Have you wondered why Aurora has different colors and what is the time line of aurora life? He explains why this is happening in this post on the Szymon Travels blog.
The dance of lights in the night sky, certainly the Northern Lights in the Northern Hemisphere or the Aurora Australis in the Southern Hemisphere, has captivated observers for centuries. The northern lights provide a breathtaking array of colors. However, their colors are not always the same, their color depends on what happens to energetically charged particles that react with atoms of elements in the atmosphere.
Cosmic dance
The interaction between charged particles?mainly protons and electrons?ejected from the sun during solar storms and the gases in our atmosphere is responsible for the aurora’s color pallet. The solar wind, a stream of charged particles that continuously emanates from the sun, carries these charged particles to Earth.
The Green Aurora Color
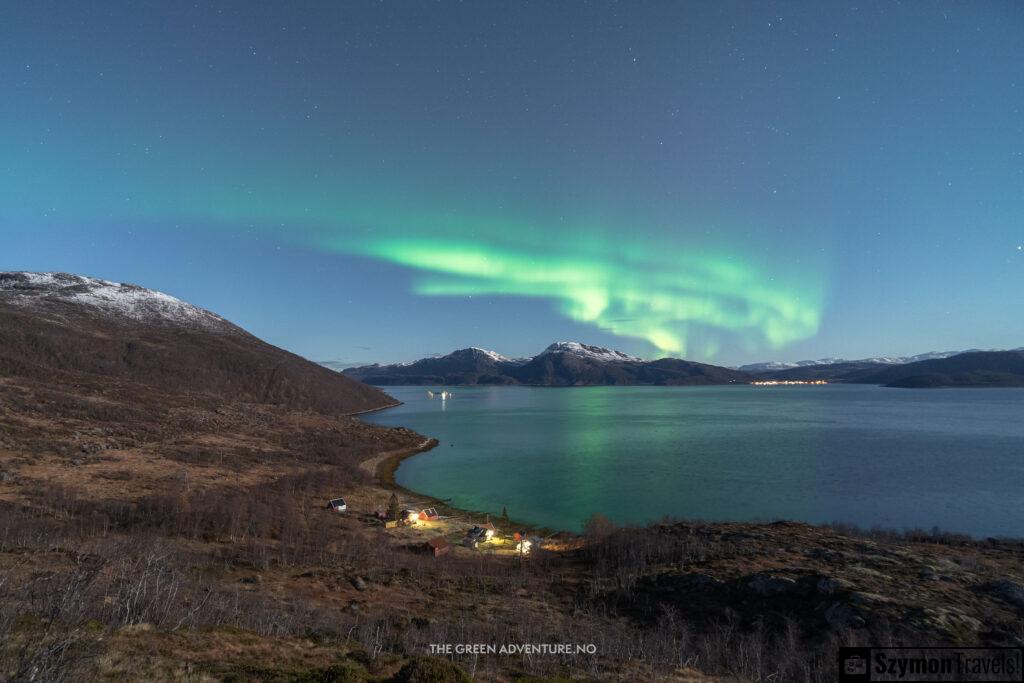
Dominating the Aurora: Green is the most prevalent color in the aurora, and its origin can be traced to the interaction between energetic electrons and oxygen molecules in the Earth’s upper atmosphere, at altitudes ranging from 100 to 300 kilometers. When these high-speed electrons collide with oxygen, they transfer energy to the molecules, prompting them to release light in the green part of the spectrum.
The Red Aurora Color

A Deeper Dive: Red hues in the aurora are more elusive and are typically observed at higher altitudes, around 200 to 300 kilometers. This distinctive color emerges when solar particles collide with high-altitude, low-density oxygen. The greater altitude and lower density cause the emitted light to shift towards the red end of the spectrum.
The Violet Aurora Color
A Symphony of Nitrogen and Oxygen: The aurora also unveils shades of violet or purple, a result of interactions between charged particles and a mix of nitrogen and oxygen molecules at even higher altitudes. This ethereal coloration adds complexity to the already enchanting celestial spectacle.
The Blue Aurora Color
Into the Depths of Earth’s Atmosphere: Blue auroras, although rarer, offer a unique and striking display. This color is typically associated with the interaction between charged particles and nitrogen molecules at lower altitudes. The scarcity of blue hues adds an extra layer of mystery to the aurora’s repertoire.
Beyond Colors
The Variables: The specific colors observed during an aurora display are influenced by various factors, including the type and energy of charged particles, altitude, and the composition of gases in the Earth’s atmosphere. Understanding these factors is crucial to unraveling the full spectrum of auroral colors.
Time line
T-Minus 0 to 2 Days: Solar Event (CME or Solar Flare):
A solar event, such as a coronal mass ejection (CME) or a solar flare, occurs on the Sun’s surface.
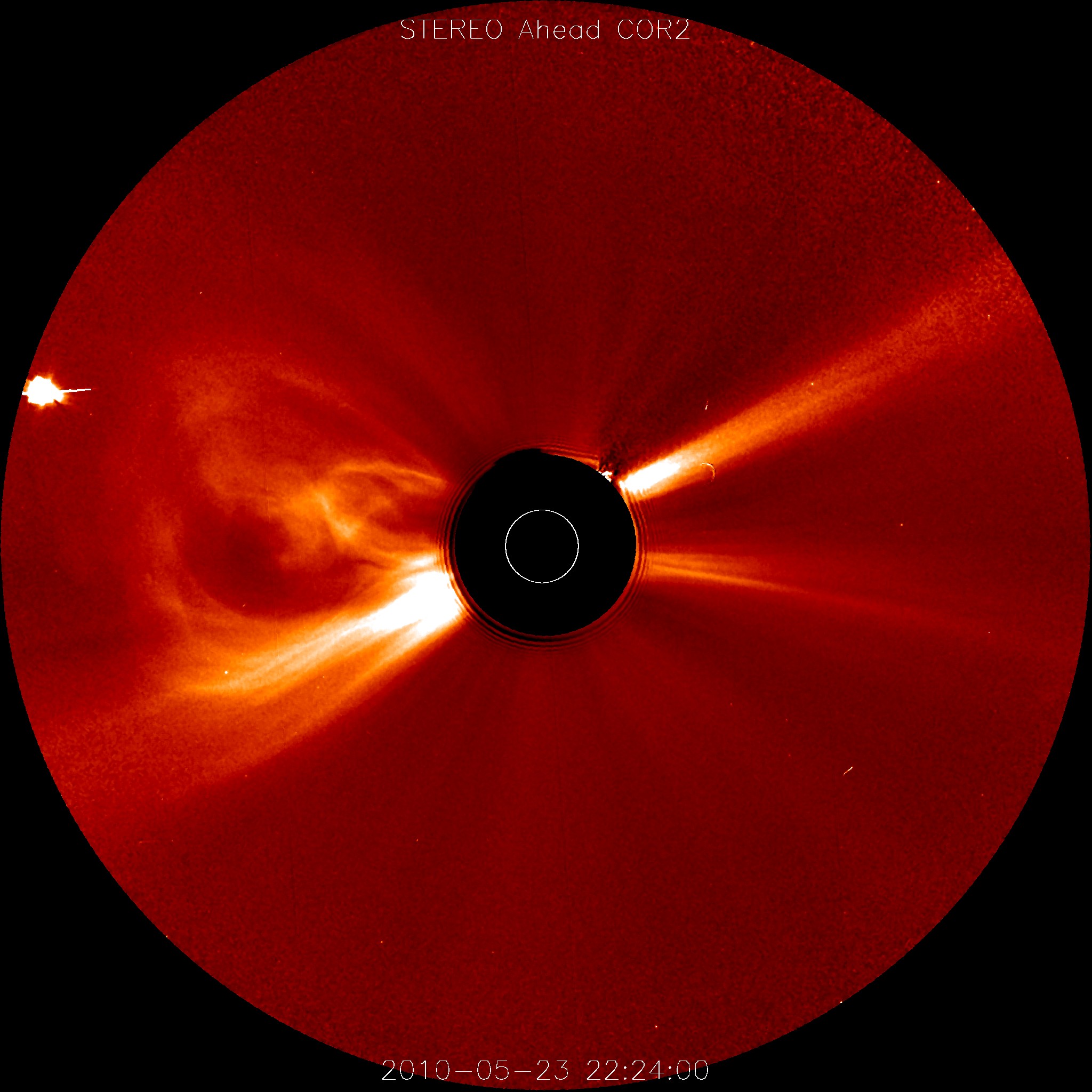
22:24:00 UT – May 23, 2010
T+1 to 2 Days: Travel through Space:
Charged particles released during the solar event travel through space as the solar wind.
T+2 Days: Arrival at Earth:
The solar wind, including charged particles, reaches Earth.
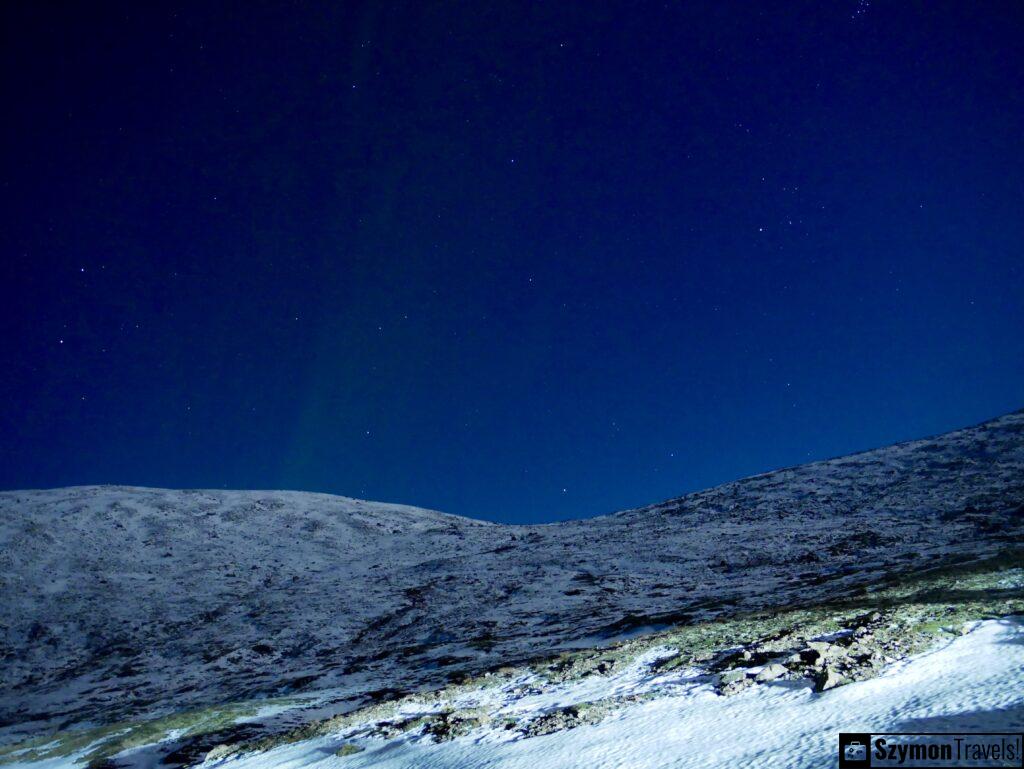
T+2 to 2.5 Days: Interaction with Earth’s Magnetosphere:
Charged particles interact with Earth’s magnetosphere, the region influenced by Earth’s magnetic field.
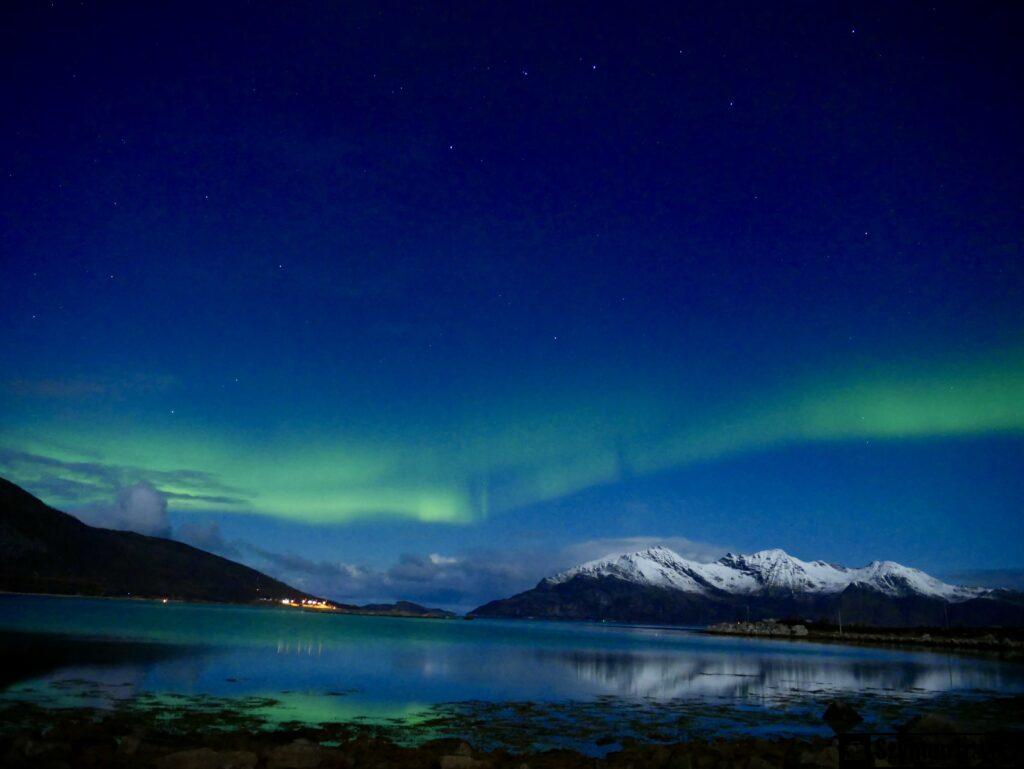
T+2.5 to 3 Days: Entry into Earth’s Atmosphere:
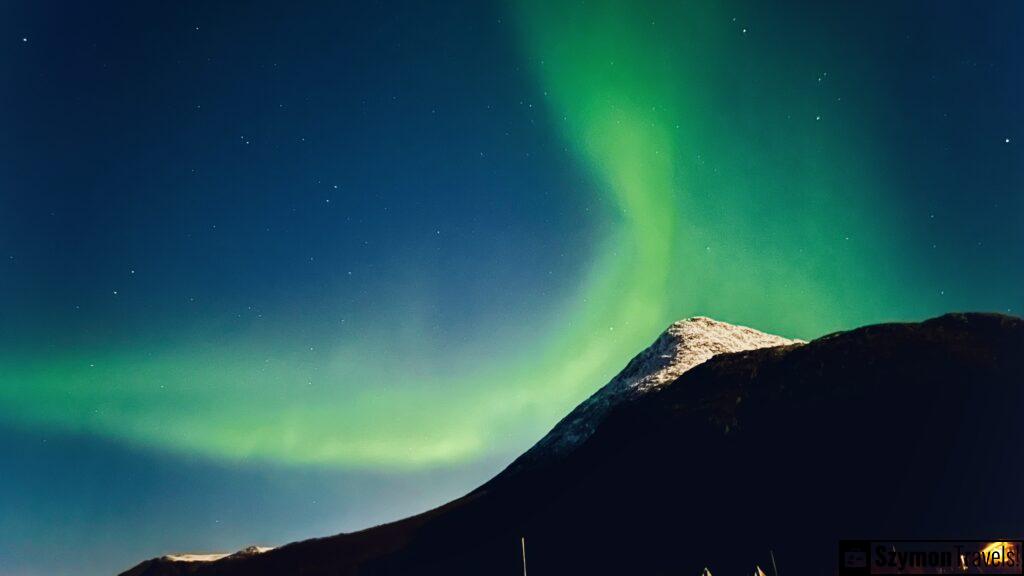
Some charged particles penetrate the Earth’s atmosphere, especially near the polar regions.
T+3 Days and Onward: Visible Aurora:
The interaction between charged particles and gases in Earth’s atmosphere leads to the emission of light, creating the visible display of the aurora.
Where else is the aurora seen in the solar system?
- Jupiter: Jupiter, the largest planet in our solar system, has a strong magnetic field and a powerful magnetosphere. Its auroras are produced by charged particles, mostly electrons, interacting with the planet’s atmosphere. Jupiter’s auroras are significantly more energetic and larger than those on Earth.
- Saturn: Similar to Jupiter, Saturn has auroras near its poles. The source of charged particles is primarily from the planet’s magnetosphere and its moon Enceladus, which expels water vapor into space.
- Uranus: Auroras have been observed on Uranus, although they are less well-studied compared to those on Jupiter and Saturn. Uranus has a tilted magnetic field, and its auroras may be influenced by the planet’s unusual axial tilt.
- Neptune: Neptune, the farthest known planet in our solar system, also exhibits auroras. Like Uranus, Neptune’s auroras are associated with its tilted magnetic field and interactions with charged particles.
- Mars: While not exactly the same as auroras on Earth, Mars has a phenomenon known as “nightglow.” This is caused by the recombination of atoms and molecules in the Martian atmosphere at night, emitting faint light. It’s not a direct result of solar wind interaction with Mars’ magnetic field, as Mars has a very weak magnetic field.
- Io (Moon of Jupiter): Io, one of Jupiter’s largest moons, exhibits auroras due to its interaction with Jupiter’s magnetosphere. The volcanic activity on Io releases sulfur dioxide gas, which becomes ionized and contributes to the auroral display.
A more scientific approach
The solar wind is a stream of charged particles released from the upper atmosphere of the Sun, known as the corona. This plasma consists mainly of electrons, protons and alpha particles with kinetic energy between 0.5 and 10 keV1. The solar wind is observed in two basic states, referred to as the slow solar wind and the fast solar wind, although their differences extend far beyond their speeds. Near Earth space, the slow solar wind is observed with speeds of 300?500 km/s, a temperature of about 100 MK, and a composition that is close to the corona1.
The magnetic field of the solar wind can be measured in three components, Bz, Bx, and By. Bx lies along the Sun-Earth line, with Bz and By defining the vertical plane (clock face). The clock angle of the solar wind is the angle formed from the vector sum of By and Bz2.
More particles in the solar wind mean more chances for the aurora, as more particles collide with Earth?s magnetosphere.
Pages that are very useful
- [SPDF – OMNIWeb Service]: This page contains hourly data on the magnetic field and plasma of the solar wind near Earth, as well as on energetic proton streams (>1 to >60 MeV) and indices of geomagnetic and solar activity.
- [NSRDB: National Solar Radiation Database]: This database contains full series of hourly and half-hourly values of meteorological data and the three most commonly measured solar radiation measurements: global horizontal, direct normal, and diffuse horizontal.
- [Real Time Solar Wind – NOAA / NWS Space Weather Prediction Center]: This page contains real-time data on the solar wind.
Source:
(1) Solar wind – Wikipedia. https://en.wikipedia.org/wiki/Solar_wind.
(2) SWS – Solar Conditions – Solar Wind Speed – Bureau of Meteorology. https://www.sws.bom.gov.au/Solar/1/4.
(3) The solar wind | Help | SpaceWeatherLive.com. https://www.spaceweatherlive.com/en/help/the-solar-wind.html.